MyTop Five
Backyard Multi-Band Wire HF Antennas by L. B.Cebik, W4RNL (SK)
My personal selection of the top 5 HF wire antennas for the backyard and for multi-band operation. Being a personal selection, there is no reason why your list should not be different from mine. But, along the way, I shall explain why I selected the 5 antenna types that I am including, giving you my views on both their advantages and their limitations. My list is simple and in no particular order.
1. The broadside doublet(s)
2. The dipole-doublet(s)
3. Fanned dipoles
4. The hohpl–horizontally oriented and polarized loop
5. The inverted-L
In order to make sense of what we say about each type of antenna, we need a point of reference. Since virtually all of the antennas will be horizontal, the logical baseline to use for comparisons is the resonant 1/2-wavelength dipole.So let’s review its characteristics.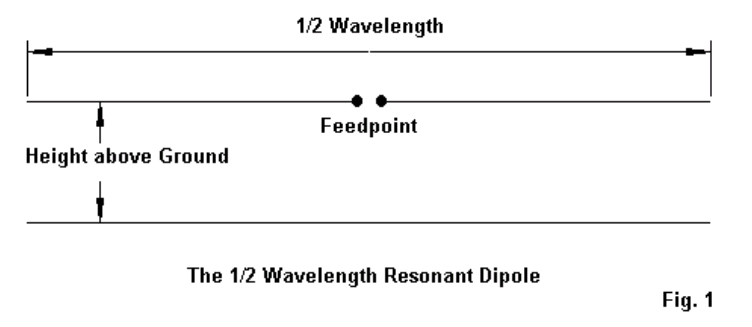
The 1/2-Wavelength Center-Fed Resonant Dipole The antenna that we loosely call the dipole is actually a 1/2-wavelength center-fed resonant or nearly resonant dipole. We usually construct it fromAWG #14 or #12 copper or copperweld wire for the lower HF bands, and we may use bare or insulated wire. Often, we mislabel multi-band doublets as dipoles because the antenna is about 1/2-wavelength at the lowest frequency of operation. But to be strictly correct, that antenna is a dipole only at the lowest frequency of use.
Fig.1 shows the two essential dimensions of a real dipole. Since we tend to feed the dipole with coaxial cable, we are concerned with the antenna length and resonance. In other words, we want a good match between the coax and the antenna feedpoint However, we also need to be equally if not more concerned with the antenna’s height above ground. The old adage, “The higher, the better,” arose from the use of wire antennas on the lower HF bands, where we generally could not achieve even a height of 1 wavelength.
Table 1.
Approximate Lengths of a Wave in Feet
Band Frequency Length Band Frequency Length
meters MHz feet meters MHz feet
160 1.8 546 20 14.0 70
80 3.6 273 17 18.1 54
75 3.9 252 15 21.0 47
60 5.37 183 12 24.95 39.5
40 7.0 140.5 10 28.2 35
30 10.1 97.5
Table 1 serves as a reminder of how long a wavelength is on each of the HF amateur bands. For most backyard antennas, the average ham is lucky to achieve an antenna height of 1 wavelength on 10 meters, while the truly fortunate operator may get his wire to 1 wavelength on 17 or 20 meters. Every horizontal antenna is subject to essentially the same general phenomena that affect horizontal dipoles in terms of their height above ground. The lower the antenna as a fraction of a wavelength, the lower will be the overall gain and the higher the elevation angle of the radiation. Fig.2 illustrates the principle for a dipole placed at 1/4, 3/8, 1/2, and 1 wavelength above average ground. Unlike vertical monopoles, horizontal wires do not change their gain or elevation angle significantly with
changes in soil quality.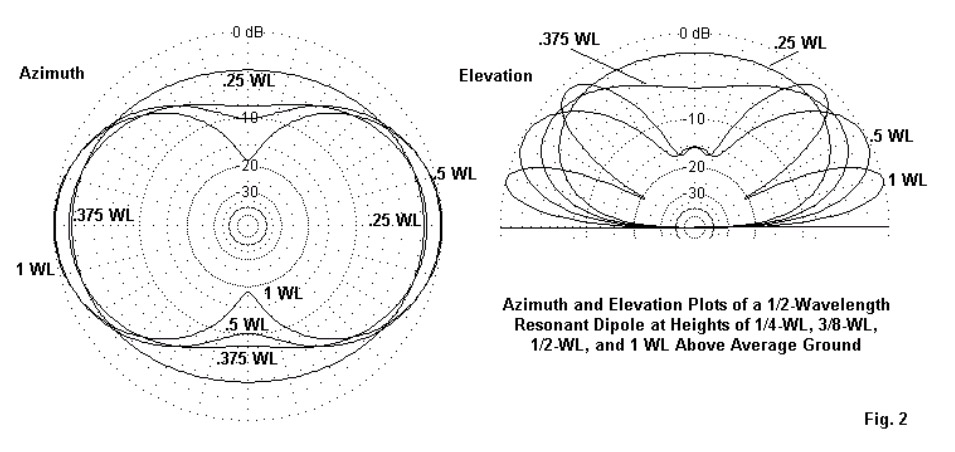
The elevation plots on the right show that the lower we place a dipole, the higher the angle of radiation, a fact that limits our effective range of communications under normal propagation conditions. The azimuth patterns on the left not only show the reduction of gain with a reduction in height, but as well the change in pattern shape. As we reduce the height of a dipole, its figure-8 shape at 1-wavelength devolves into a simple oval at a height of 1/4-wavelength. What applies to the dipole will generally but not without some exceptions, apply to any horizontal wire antenna relative to its height above ground at the frequency of operation. When it comes to height, think wavelengths, not feet! 1/2-wavelength resonant center-fed dipoles have many other interesting characteristics, but the ones that we have noted will guide us while we explore the top 5 multi-band backyard wire antennas. We shall also be setting aside our coaxial cable in favour of parallel feedline to an antenna tuner (ATU) or, as some British writers prefer, an antenna system tuning unit (ASTU). Consider the ATU to be a lifetime investment.
1. The Broadside Doublet(s): The broadside doublet is a simple multi-band doublet with a 4:1 frequency range for the desired characteristic. Fig. 3 shows the general outline.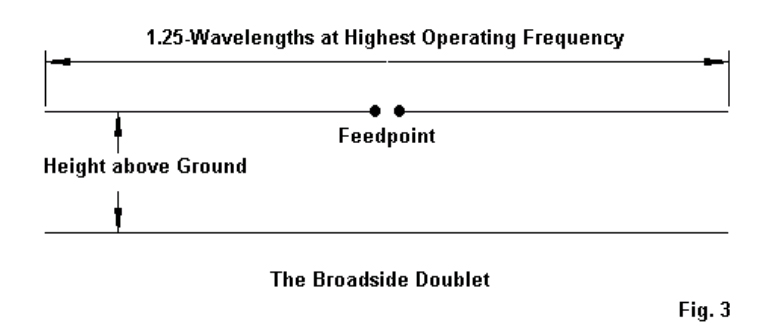
In principle, the doublet is physically no different from a dipole. However, electrically, it is
significantly different. First, we feed it with parallel transmission line to an antenna tuner, because the feed point impedance varies greatly as we change operating frequencies from one band to the next. Second, we select the length so that the antenna will show a bi-directional pattern broadside to the wire on all of the bands included. Note that the length makes the antenna an extended double Zepp at the highest frequency. With an antenna tuner, the antenna will operate above its highest included frequency, but the pattern will breakup into multiple lobes. Table 2 provides the most convenient lengths and the bands included.
Table 2. Broadside Doublet Lengths and Amateur Band Coverage
Length (feet) Bands covered
44′ 10, 12, 15, 17, 20, 30, 40 meters
66′ 15, 17, 20, 30, 40, 60 meters
88′ 20, 30, 40, 60, 80 meters
The chief advantage of the broadside doublet is that you always know the directions of your radiation or your most sensitive reception. A second advantage is the antenna’s simplicity for a 4:1 frequency range with the bi-directional characteristic. A third advantage, which we shall note shortly, is the flexibility of the antenna in forming wire arrays having different characteristics. With every set of advantages come one or more disadvantages. First, the antenna requires a wide-range ATU, since the impedance varies greatly from band to band.Since the exact values that will appear at the tuner terminals will vary with both the antenna height and the length and characteristic impedance of the parallel transmission line, I shall not provide specific numbers. Second, the gain goes down with frequency. The broadside doublet has its highest gain at the highest frequency. The gain drops a bit with each move to a lower band, while the pattern broadens. Fig. 4 shows the patterns overlapped in free-space models. However, remember that as we go down in frequency, the antenna will have a height that is a smaller fraction o fa wavelength. Hence–like the dipole–we can expect a further reduction in gain and a more significant increase in the elevation angle of maximum radiation. Hence,the higher you can place the antenna, he better will be its performance.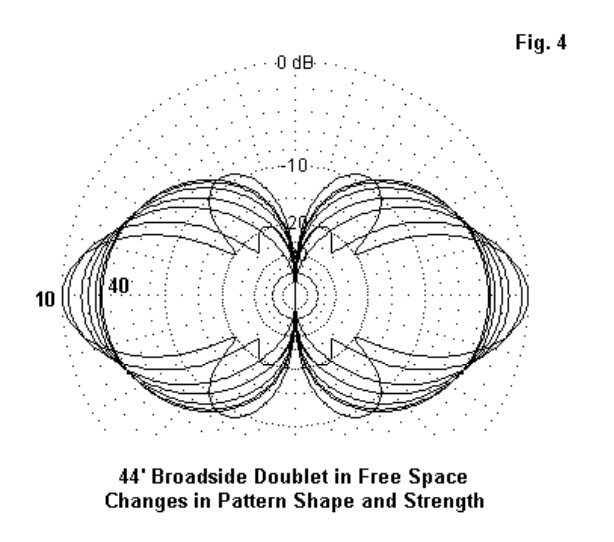
Part of the flexibility of the broadside doublet stems from the ease of covering the full horizon by adding only one more support. See Fig. 5.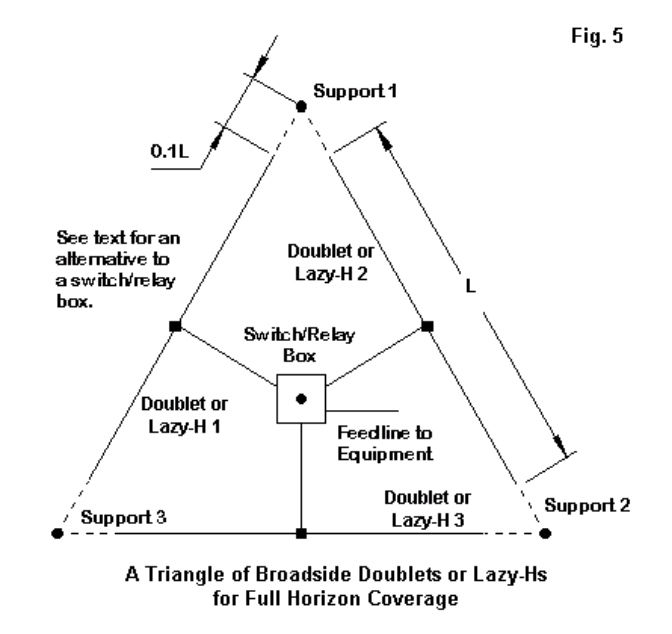
We can easily form a triangle of doublets. The triangle need not be perfect, so you can adjust it to aim more exactly at your favourite communications targets. With only a bit of end space(about 10% of the wire length), the inert wires will not materially affect the operation of the one in use. The only caution that you need to observe is to keep the feedline lengths identical. This caution applies whether you use a fancy switching box at the center of the array or whether you bring three separate and well-spaced lines to the shack entry point and do your switching indoors. Equal line lengths will mean that you do not have to do major retuning when switching from one antenna to the other. Hence, you can easily determine the most effective antenna for an incoming signal just by switching through the 3 antennas. Note that the triangle refers to either doublets or lazy-H antennas. That is part of the flexibility of the broadside doublet system. We can make lazy-H arrays using any of the listed element lengths and cover the same set of bands–but with more gain. Fig. 6 the outlines of a lazy-H.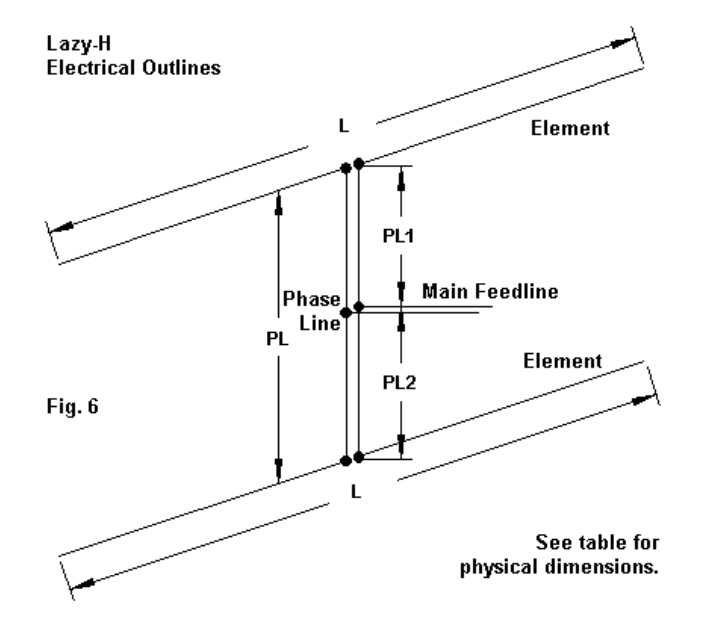
The lazy-H is simply 2 broadside doublets fed in phase. We need the center main feed point to ensure that the lines to both wires are the same length and therefore give us the same current magnitude and phase angle at both element feed points. So PL1 and PL2 are 1/2 of PL. The total length of the phase line assembly can be longer than the spacing, but for most installations, they are the same. The ideal spacing is 1/2 of L, the element length. Hence, the spacing is 5/8-wavelength at the frequency where the element is 1.25 wavelengths. This spacing provides maximum gain. You can reduce the spacing somewhat,but every reduction reduces the gain on all bands. The ideal lazy-H will net you almost 3-dB gain on the highest bands. There will be a slight reduction for the lowest bands, since the spacing will no longer be optimal. As well, the lower wire gets closer to the ground as a fraction ofa wavelength when we reduce the frequency.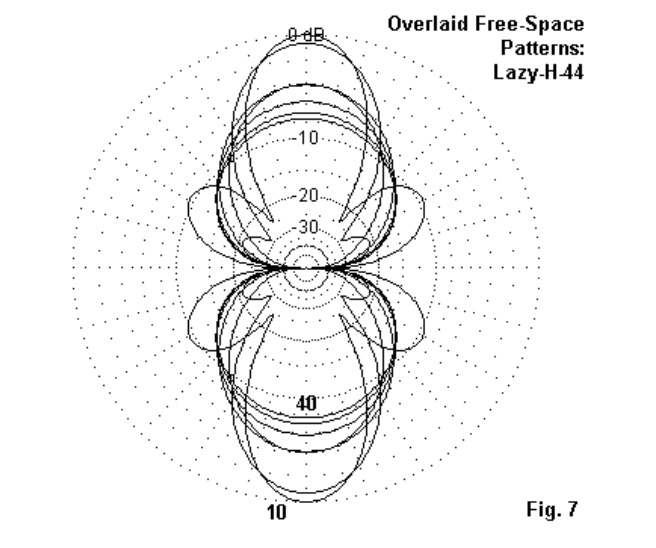
Fig.7 shows the overlaid free-space patterns to give you a basic idea of what happens to shape and strength,but remember to modify your expectations depending upon the height that you place the antenna. Getting the lowest wire at least 1/2-wavelength above ground is best,although lower heights for that wire will work. However, if that wire will be under 1/4-wavelength above ground, you maybe better off with a simple broadside doublet at the upper level. It will give you a lower radiation angle than the pair of wires. As a side note, in any set of in-phase fed antennas–whether doublets, Yagis, or whatever–the effective height of the combination will be a point about 2/3 the distance between the lowest and the highest antennas. Of course, we can make a triangle of lazy-Hs, just as we can for the basic doublet. There is a second multi-band array that we can make from the broadside doublet: an 8JK.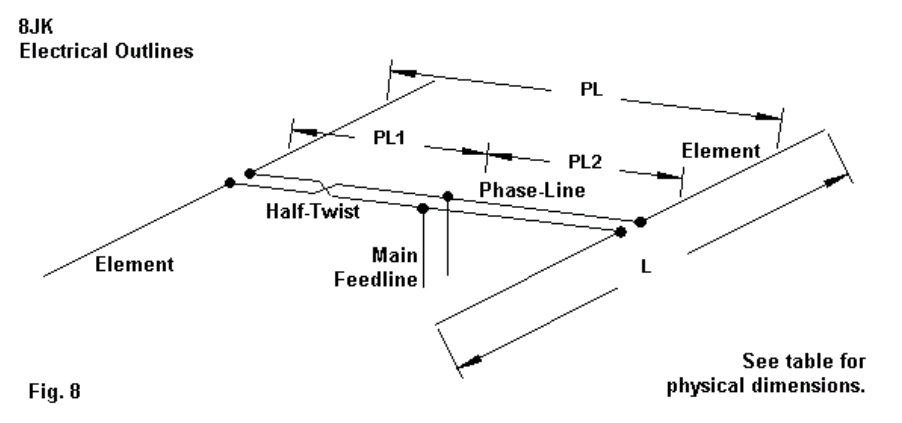
See Fig. 8 for the outline. Developed by John Kraus, W8JK, in the1930s, the antenna has undergone many variations. The versions shown here is designed for a 3:1 frequency range. It uses the broadside doublet lengths for the highest frequency,along with a total phase-line length that is 1/2 of the element length, L. However, note that when we create this end-fire array, we give one (and only one) of the phase line sections a half twist. The specific dimensions we have chosen from W8JK’s work are ones that give us an interesting pattern of gain, as shown in the free-space patterns of Fig. 9. The free-space gains are about equal on all bands. Indeed, the only factor that limits our frequency coverage is a very low impedance below the listed frequency limit.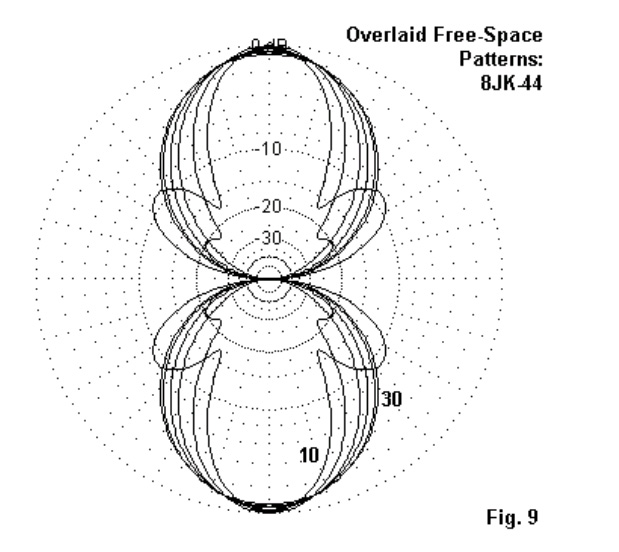
Of course, over ground, the gain will decrease as we lower the frequency,since the array will be lower as a fraction of a wavelength. Within the included bands, the gain will be much more equal from band to band than with the lazy-H. However, the peak gain will not be as high at the highest covered bands.
We may summarize the array dimensions in a simple table(Table 3). Remember that since we are using parallel feedline and an antenna tuner, broadside doublet lengths are not finicky. However, in the arrays, strive for equal lengths for each element.
Table 3. Lazy-H and 8JK Dimensions
Element Length Phase-Line Bands covered (meters)
(L, feet) Length (PL, feet) Lazy-H 8JK
44′ 22′ 10 – 40 10 – 30
66′ 33′ 15 – 60 15 – 40
88′ 44′ 20 – 80 20 – 60
2. The Dipole-Doublet(s): The dipole-doublets differ from the broadside doublets in two respects. First, rather than determining their length based on the highest frequency of use, we determine it based on the lowest frequency.In most cases, the doublet is a 1/2-wavelength dipole (approximately) at the lowest frequency. (Even the G5RV doublet is a dipole on 60 meters.) Again,since we shall use parallel feed line and an ATU, we do not have to be finicky in setting the exact length. Second, we do not give any preliminary thought to the lobe structure of the radiation pattern when we set up a dipole-doublet. Usually, we know that thepattern is bi-directional at the lowest frequency. However, we often do not think about the pattern above that frequency. As we shall see, the bi-directional patterns holds true until the antenna length as measured in wavelengths is greater than about 1.25.However, for many users, the patterns for the higher bands are mysterious. To get us started, Fig. 10 outlines the basic dipole-doublet. It chief advantages are simplicity and the ability to cover all of the HF bands above the frequency for which the wire is a 1/2-wavelength dipole. Hence, the preferred lengths are usually about 260′ for 160-meter coverage, 135′ for 80-meter coverage, and 67′ for coverage down to 40 meters. As noted, the G5RV 102′ doublet manages to be a dipole at 60 meters. The 67′ length will load on 60 meters, and the G5RV will load on 80 meters. In each case, the wire is about 1/3-wavelength,close to the limit for a center-fed wire.Below that length, the resistive part of the impedance goes too low and the capacitive reactance goes too high for most parallel line and tuner combinations to handle.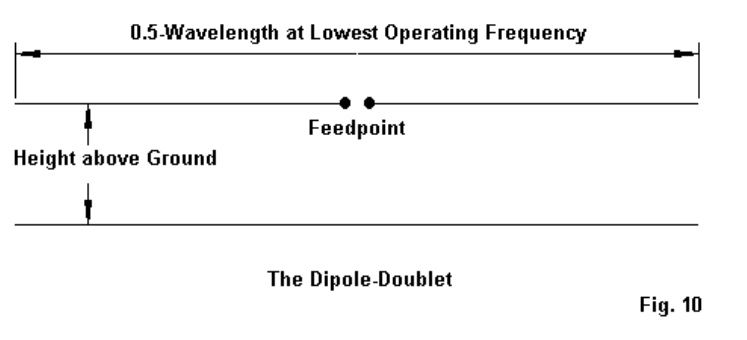
To start the process of becoming familiar with the typical patterns of a dipole doublet, examine Fig. 11. The dipole-doublet’s length in feet matters less than how long the doublet is at a given operating frequency in terms of half-wavelengths. When the length is close to an even number of half-wavelengths, we have as many lobes as we do half-wavelengths. The strongest lobe moves farther from a broadside direction as we increase frequency. As well, the beam width of the strongest lobe becomes narrower. Because the ham-band operating frequencies do not result in exact multiples of a half-wavelength, the lobe strengths will vary. But the count remains true.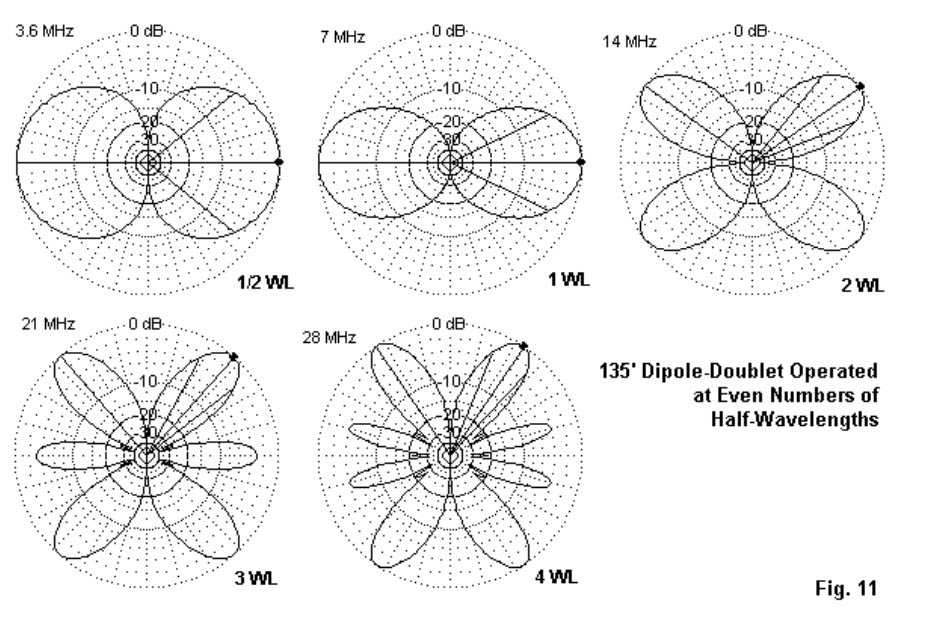
If we operate the same antenna at odd multiples ofa half-wavelength, we obtain patterns composed of both emerging lobes and diminishing lobes. So the number of lobes is the sum of the old even number of half-wavelengths and the new even number of half wavelengths. In other words, we have twice the number of lobes as we do the length in half wavelengths. See Fig. 12. Again, because the nearest ham-band frequencies are not precisely the number of half-wavelengths listed, we find some of the lobes weaker than others. However, the count remains true.
The patterns would change if we used a 102’or a 67′ doublet when referenced to specific frequencies. However, relative to the frequency at which the doublet is 1/2-wavelength long, the patterns would re-emerge as shown as the antenna approaches lengths of 1 wavelength, 3/2 wavelengths, 2 wavelengths etc. In addition, the dipole-doublet is subject to the same rules relating antenna height as a fraction of a wavelength to radiation elevation angles that we discovered for the resonant dipole. If we understand the pattern development of a dipole-doublet, we can successfully use it without disappointments. The feed point impedance will vary over a wide range. In fact, it will be very high whenever the doublet is a multiple of a full wavelength. Hence, many users prefer 600-800-Ohm ladder line so that the line is an intermediate impedance between the highest and lowest values encountered. I like the old dipole-doublets for their simplicity and their long tradition of successful use. They are also flexible. We can set up a triangle of them, but the complex patterns may not give us the full horizon coverage of the triangle of broadside doublets. There is even an old (1930s) trick that we can use with the dipole-doublet: the center-support Y. Fig.13 shows the general outlines. The sketch shows 67′ legs, comparable to a 135′ doublet.However, you can use 50′ or 35′ legs with reduced low band coverage.
The Y-doublet’s special feature is the use ofa non-conductive center support (which may be no support at all if you can devise a way to hang the center freely). Either by spacing wires from a center pole or using triangular spacers, we bring down 3 wires, one from the inner end of each leg. The down-wires form the parallel transmission line. At the shack entry point, we set up a switching system to select the pair of wires to form the transmission line for the active doublet. In most cases, it will not matter whether thethird wire simply floats or is grounded:it is centred between the 2 active wires and has
almost no current on it. The Y-doublets form 120-degree angles. This angle makes almost no difference in the pattern relative to a linear doublet. There will be some differences in the patterns on the upper bands compared to those we saw for the linear doublet. Nevertheless, you will use an A-B-C switch to determine which pair of legs provides strongest signal. In order to make radical re-tuning unnecessary,itis important to
keep the transmission line wires equally spaced in a triangle all the way to the switch at the shack entry point. The Y-doublet is one way to overcome some of the limitations of the dipole- doublet’s multiple lobes on the upper HF bands.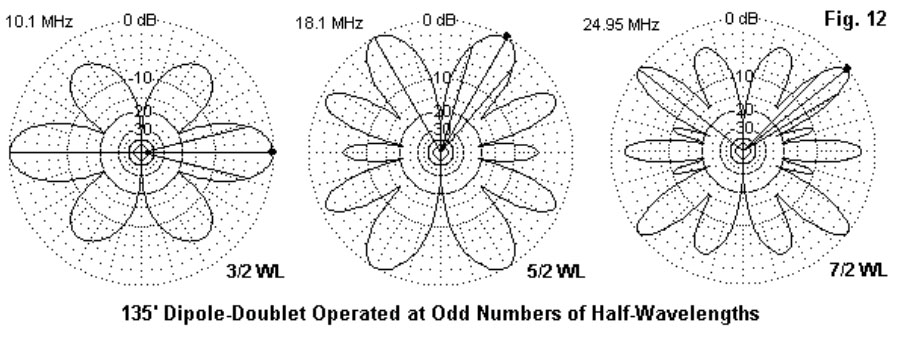
3. Fanned Dipoles: For coax lovers, mythird selection for a multi-band antenna is fanned dipoles. I have seen a myriad of designs for these antennas, some of which include up to five or 6 bands and fold the longest elements into spaghetti. These designs I do not prefer, because they have very narrow operating bandwidths and erratic patterns due to combining long and short elements that both offer low feedpoint impedances. For reliable service with decent bandwidths, I prefer to construct my fanned dipoles a couple of bands at a time so that when one band shows a low impedance, the impedance of the other band is high. As well I prefer to widely space the shorter element outer ends from the longer element and obtain a wider operating bandwidth on both bands. In short, my preference in fanned dipoles is a 2-band antenna,although I would not rule out a 3-band combination. Fig. 14 shows the general outlines with the critical dimensions noted. Ordinarily, we support the outer ends of the longer dipole and suspend the shorter dipole beneath.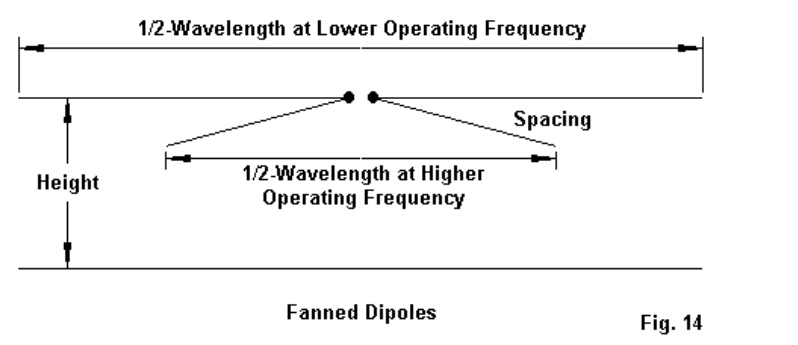
Let’s get a handle on the properties of fanned dipoles with a simple 80-40-meter combination. We shall look at two versions of the same antenna. One will droop the 40-meter dipole 10′ below the 80-meter dipole. The other design will place the 40-meter outer ends 1′ below the low-band dipole. The 80-meter dipole is set for 3.6 MHz, while the 40-meter dipole is set for 7.1 MHz. Table 4 gives us the dimensions and the performance of the array on each band with the 80-meter dipole 50′ above average ground.
Table 4.
Fanned 80-40-Meter Dipole Dimensions and Modelled Performance at 50′
Frequency Length Gain TO Angle Feedpoint Z
MHz feet dBi degrees R+/-jX Ohms
Wide-Spaced Version
3.6 130.4 6.63 86 61 – j 1
7.1 67.0 4.82 44 54 – j 0
Close-Spaced Version
3.6 130.4 6.80 88 61 + j 2
7.1 75.3 4.85 40 58 + j 2
Although there is no significant difference in performance at the two design frequencies between . the wide and the close dipoles, we certainly can see a difference in the length of the 40-meter dipole. The wide-spaced version shows a length that approximates the length of an independent 40-meter dipole. However, the close-spaced version requires a much longer 40-meter element. Remember that the close spacing is still 1′, which is wider than some published and commercial designs.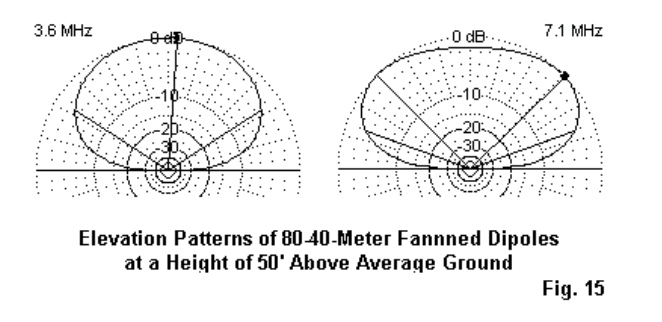
Fig. 15 shows the elevation patterns for the two bands. Like all horizontal antennas at low heights, neither pattern is ideal. The antenna on 80 meters is below 0.2 wavelength, and it only achieves 0.36 wavelength on 40 meters. As with all of the horizontal wire antennas, it could benefit from additional height.
We cannot see much difference in performance between the two versions of the antenna on the design frequencies, but we did note the 40-meter dimension change to obtain that performance. The 80-meter length did not change as we altered the spacing of the 40-meter ends from the 80-meter wire. Obviously, in a fanned dipole arrangement, we expect the shorter wire to undergo more change than the longer one. The question is how we can sample the long-wire stability and the short-wire variability.
One way to see the difference is to examine the 50-Ohm SWR curves for the two versions of fanned dipoles. Since the 80-meter wire remained stable, we would expect the SWR (as a measure of changes in resistance and reactance also to remain stable. Fig. 16 tells the story.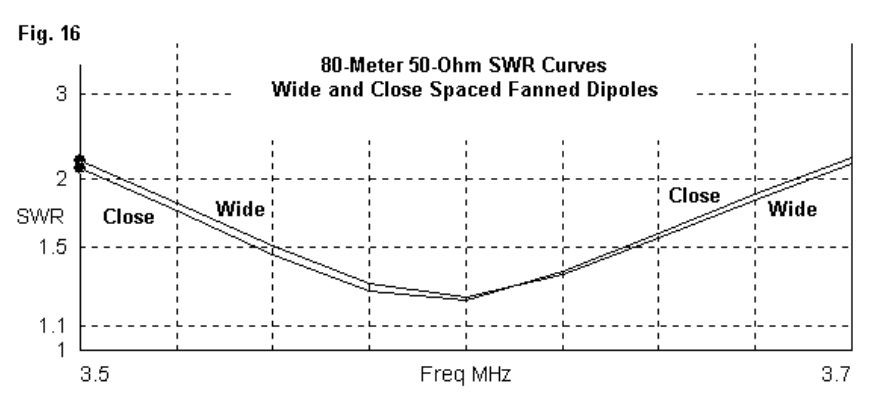
The 40-meter 50-Ohm curves stands in stark contrast, as revealed by Fig. 17. The wide-spaced version of the antenna covers over 2/3 of the band. The close-spaced version barely handles 100 kHz. While the narrower bandwidth may be satisfactory for some operational needs, it also indicates that pruning the close-spaced 40-meter dipole to length is likely to be a somewhat ticklish task.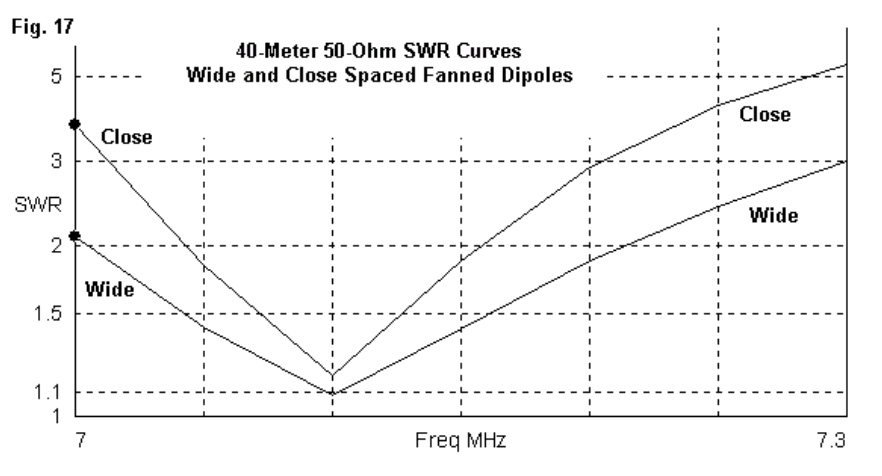
To understand why closing the space between the dipoles shrinks the usable passband of the higher-frequency dipole, we should make at least one more probe into the operation of the antennas. Modelling software gives us a look at the current distribution along the dipoles. Let’s compare the currents on both versions of the array.
Fig. 18 shows in the curves on the left the relative currents on the wires during 40-meter
operation. Notice that, despite the high impedance of the 80-meter dipole, there remains a low but significant current on the wire. Even with wide spacing and a predominance of current on the 40-meter wire, the two dipoles do not achieve the kind of independence that casual fanned-dipole theory suggests. When we move to the right and examine the closed spaced version ofthe array, we see a considerable increase of current on the 80-meter dipole. The closer that we space the two dipoles,the higher the current on the longer one. The higher the current that we find on the longer dipole, the narrower will be the operating passband of the shorter dipole and the more painful will be the job of setting its proper
length. As well, we are likely to find that a set of lengths that is right for one antenna height is not right for a different height.
Fanned dipoles have served me well over the years, but only when I restricted the number of bands covered and when I separated the shorter dipole ends as far as feasible from the longer wire. As well, a 2:1 frequency ratio has tended to yield the most successful antennas with the widest operating bandwidth. Still, do not count the fanned dipole out when it comes to flexibility. I once tied a 10-meter vertical dipole to a horizontal 15-meter dipole with good success. One might even use close spacing (and patient pruning) to set up a combination for 12 and 17 meters or for 17 and 30 meters, where operating bandwidth is less of a question. However, I likely would steer away from combinations like 40 and 15 meters or 30 and 12 meters.
4. The HOHPL–Horizontally Oriented and Polarized Loop: The horizontal loop is subject to several misconceptions. Two of the most popular are that 1. The longer I make the loop, the more gain I get, and 2. The loop gives me omni-directional coverage on all of the HF bands. Basically, if you want more gain, then place the antenna higher. Moreover, even if you create a perfect circle, your pattern will not be circular on almost any band. Nevertheless, the loop is a good multi-band antenna easily fed with parallel transmission line and an ATU.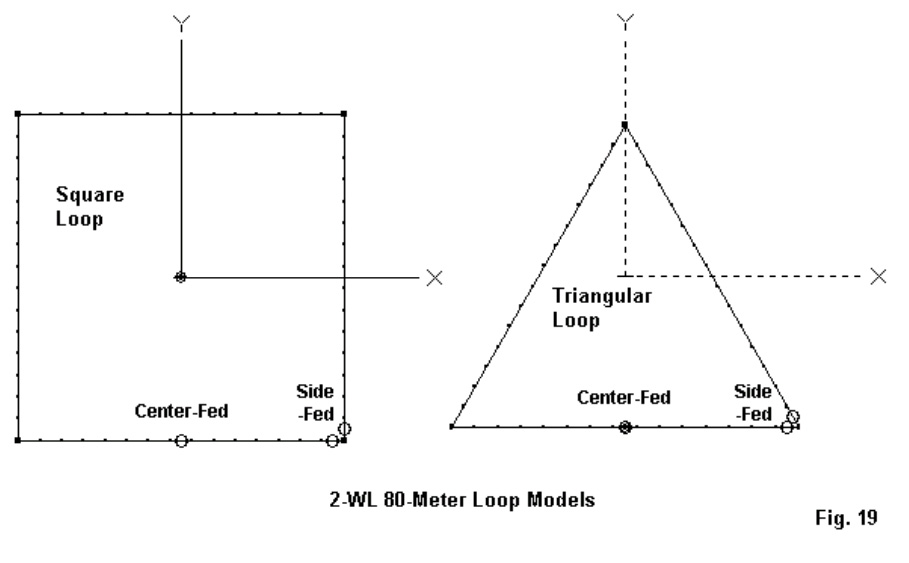
Fig. 19 shows the outlines of the two most popular shapes for a horizontal loop: the square and the triangle.Almost any other closed shape–regular or irregular–is possible. Most polygons with more sides tend to act like squares, so the contrast between the square and the triangle become good guides on what to expect from a loop strung along the perimeter trees in an average yard.Each loop shows 2 (different) feedpoints: a mid-side location and a corner location. These two points tend to coincide with the most convenient installation points from whichto runthe parallel feedline fromthe antenna to the
ATU. You can select an alternative position and nothing evil will happen. However, the patterns will not be as regular as the ones that we shall use for demonstration purposes.
I prefer to use a 2-wavelength loop at the lowest frequency ofoperation. In fact, our
demonstration loops will be 560′ loops with 80 meters as the lowest band. Loops have a peculiarity.
If we make them about 0.75-wavelength or smaller, they tend to radiate off the loop edge. If we make them close to 2 wavelengths or larger, they also tend to radiate off the edge. However,if we make the loop 1-wavelength–or thereabouts–at the frequency of operation, then it radiates broadside to the loop. Hence, a horizontal 1-wavelength loop becomes a good NVIS antenna, as our 80-meter 2-wavelength loop would become on 160 meters. Incidentally, we shall place the demonstration loop 70’above ground simply for the exercise.70′ is about 1/4-wavelength on 80 meters but 2 wavelengths on 10 meters. The phenomena of changing planes of radiation as we enlarge a loop will explain why my list of
the top five multi-band antennas does not include any vertically oriented loops. Lets start with a 1-wavelength loop.It does fine on the band for which it is cut. However, by the time we double the frequency of operation,the loop is radiating off the edge, producing mostly high-angle radiation. Such loops will make contacts, but not as well as a horizontal loop.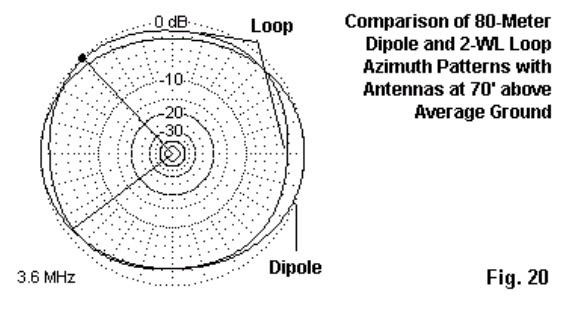
On the lowest band of operations, we do not choose the loop for gain. As shown in Fig. 20, there is very little gain difference between the loop and a resonant dipole at the same height. The mid-side-fed square loop used to generate the loop part of the pattern is not even significantly more omni-directional than the dipole pattern. However, at their lowest operating frequencies, loops tend to show a lower radiation angle than a low dipole. In the case that uses antennas at the 70′ level, the dipole’s take-off angle is 58 degrees, but the loop’s angle is between 44 and 50 degrees, depending upon the loop shape and the feed point position. This advantage is useful at the lowest operating frequency, but it does not last as we increase frequency. By the time we double the operating frequency,the take-off angle for loops tracks well with the take-off angles for doublets at the same height.
Loop antenna shape and the position of the feedpoint do make a difference to the antenna’s pattern and performance.
Fig. 21 shows the 3.6-MHz azimuth patterns for the two loops (square and triangular) with both corner and mid-side feed points. The notation FP on the plots shows the relative position of the feed point to the development of the plot. The triangular plots are–relative to the feed point position–more alike than the two plots for the square loop. In both triangle cases, the direction of the main lobe crosses the feed point and a point in the middle of the opposite side. The main difference is a reverse in the slight gain advantage. For the corner-fed triangle, maximum gain is away from the feed point, while in the mid-side version, gain is more toward the feed point. The squares, however, show a more distinct pattern difference, depending upon the feedpoint position. The corner feedpoint produces a nearly circular pattern, while the mid-side feedpoint yields a 4-lobe pattern.
Although there are no major differences in gain, Table 5 presents the modelled maximum gain values and take-off angles for the 4 loops on various HF bands, using our basic 560’loops at 70′ above average ground. Because we shall use an ATU, the feedpoint impedance data is not especially useful here.
Table 5. Modelled Performance of 560′ Horizontal Loops at 70′ on Selected Amateur Bands
Note: Performance shown as Maximum Gain (dBi)/TO Angle (degrees).
Loop Square Square Triangle Triangle
Frequency Corner-Fed Side-Fed Corner-Fed Side-Fed
3.6 5.9/50 6.7/44 7.1/48 6.4/45
7.0 10.6/27 9.9/26 9.7/28 10.4/28
14.0 12.9/12 11.6/12 13.9/13 11.2/13
21.0 14.6/9 14.3/9 13.4/9 12.4/9
28.0 15.1/7 13.6/7 14.1/7 11.0/7
I have inserted Fig. 22 immediately following the chart of modelled gain values so that you will not make too hasty a decision on which loop to select. It shows the patterns on 40 through 10 meters that produce the gain figures in the chart, at least for the square loops. Above 40 meters, we discover patterns with many lobes. The higher we go in frequency,the more lobes we encounter and the more variable we find the lobe strength. As a general rule of thumb with simple wire antennas, the higher the gain of a lobe, the narrower its beam width. So we obtain maximum gain only over as mall target communications arc. In these patterns, dots serve to locate the feed point position on the loop relative to the pattern.
Fig. 23 provides the corresponding patterns for the triangular loop. On 80 through 20 meters, the loop produces patterns that have a distinct axis on the line formed by the feedpoint and the opposing loop position. Above 20 meters, the patterns become as individual as snowflakes, each very regular but distinct from the other patterns. When we combine the patterns of the three plot figures together, we may finally come to understand that on the upper HF bands, horizontal loops do not produce patterns that are more omni-directional than doublets. The patterns are simply different in the relative positions o the lobes and nulls. As well, the chart shows that on the upper HF bands, the radiation angles are similar to those of simple wire doublets.
So why choose a horizontal loop as one’s multi-bandwire antenna? One good reason is the improvement in radiation angle on the lowest frequencies of operation. A second good reason is because you have the perimeter supports already growing in your yard.A third reason is because closed loops tend to be less prone to the build up of static charge relative to doublets with un-terminated ends. This feature does not guarantee immunity from all noise sources. Nor does it guarantee immunity from the hazards or lightning and associated thunder storm dangers. A fourth reason is becauselarge closed loops tend tohave less high-angle radiation. What may be more important, this fact means less receiving sensitivity to high-angle sensitivity to QRM andQRN from closer-in sources. Fig. 24 compares the 21-MHz elevation patterns for a simple 135′ linear doublet and a 560′ triangular loop with its feedpoint in the middle of oneside. Although the exact shapes of the
15-meter elevation patterns will vary from one loop to another in the set that we have been examining, they all share the general property of having less high-angle radiation. The loop has several advantages that recommend it as a multi-band antenna,if you can live with the upper band patterns, if you have room for the array, if you can find the wire and the supports, and if you are prepared for the maintenance that a long stretch of wire requires. All those “ifs,” of course, represent the disadvantages of the horizontal loop.
5. The Inverted-L: In principle,the inverted-L is any antenna that physically looks like a tipped-over letter L. Electrically, it includes not only tuned systems with radials, but as well, any sloping wire that runs from an antenna tuner at ground level to some higher end-point. (A sloping wire has essentially the same vertical and horizontal radiation components as the more formally positioned L.) What we used to call a random length, end-fed wire belongs in this group as much as the 160-meter inverted-L with 64 radials at its base. In more practical terms, the average backyard antenna builder is unlikely to lay down a major radial field for the most efficient 160-meter operation. The portion of the antenna above ground is no problem, since it involves running a wire up as high as available supports will permit and then running the remainder horizontally. The radials are the major hindrance to using the inverted-L. So let’s reduce the radial field to a mere 4 radials, each 1/4-wavelength long at 160 meters, that is, about 135′. With this reduced field,let’s assume that we have 50′ of vertical support. Then an all-band inverted-L will look something like the left-hand portion of Fig. 25,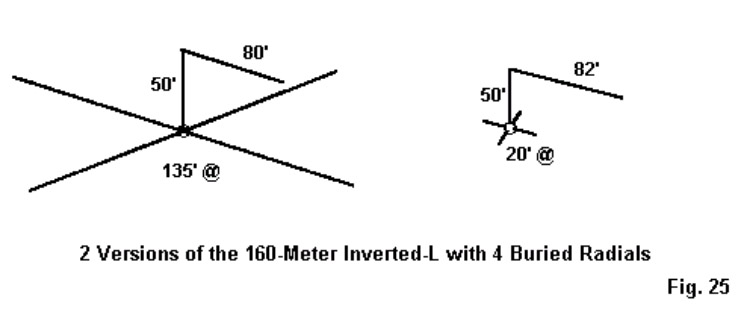
To achieve resonance on about 1.85 MHz with AWG #12 wire throughout, we need an 80′
horizontal run. Incidentally,in my model, the radials are buried 6″ deep in the ground, but the exact burial depth is not critical.Now let’s add a second dose of reality. Many hams have backyard filled with gardens, children’s toys, garages, etc. Hence, we find an unwillingness to commit to more than short radials. So the right-hand side of Fig. 25 shows the same set-up with 4 20′ radials. The horizontal section of the antenna needed a 2′ extension to restore resonance at 1.85 MHz.Now let’s compare performance (Table 6).
Table 6. Inverted-L Performance with Full and Short Radial Systems
Antenna 135′ Radials 20′ Radials
Frequency Gain TO Angle Feed Z Gain TO Angle Feed Z
MHz dBi degrees R+/-jX Ohms dBi degrees R+/-jX Ohms
1.85 -2.2 29 38 – j 2 – 2.6 29 47 + j 2
3.6 3.5 84 4500+ j1750 3.8 84 5200- j150
7.0 4.0 35 700 + j 750 4.0 35 900 + j 800
14.0 5.2 22 300 + j 300 5.3 23 350 + j 350
21.0 6.0 13 200 + j 80 5.8 13 250 + j 200
28.0 7.7 10 200 – j 100 7.5 10 200 + j 30
In practical terms, we find a significant performance difference only on 160 meters. The #12 inverted-L is under ideal conditions about 2 dB less effective than a full size vertical monopole. When we shorten the radials, we lose another half-dB of gain. Otherwise, the two systems are roughly equivalent for all-band operation. The short radials and longer horizontal section of the smaller system do raise the impedance values, but if a tuner will handle one set,it will also handle the other.
Fig. 26 shows the patterns for 160 meters, with the antenna orientation marked. Note that the horizontal section offsets the vertical monopole pattern away fromitself bya small amount. Also note that there is still significant current in the horizontal portion ofthe antenna. This shows up as the figure-8 horizontallypolarized component of the pattern that is about 10-dB down from the vertically polarized component. As we move above 160 meters, we can discover one reason why many inverted-L users think of the antenna as a good (even if not perfect) general communications antenna.
Fig. 27 provides patterns for selected hambands, with the frequency and the TO angle noted for each azimuth plot. Relative to patterns for closed loops and linear doublets, the patterns are almost all more equally distributed around the horizon. As we increase frequency, the combination of increasingly strong horizontally polarized radiation and the remnant vertically polarized radiation tend to provide a modicum of gain in almost every direction. Only when we reach 10 meters do we find a pattern of well-defined lobes and nulls, but the nulls are not as deep as those we find with loops and linear doublets. The cost of the fuller coverage is lower maximum gain. There is no significant difference between the pattern shapes for the L with a full radial field or the L with short radials. Remember that these patterns apply to an inverted-L with a 50′ vertical section. If you bend the L at a lower height, the TO angles for 80 through 10 meters will rise, and the gain and exact pattern shape may change. However, let’s consider one more version in which the user does not lay down a symmetrical (or thereabouts) radial field. The one thing necessary to the use of the inverted-L is a good RF ground, so this user lays down 1 buried radial about 20′ long. See Fig. 28.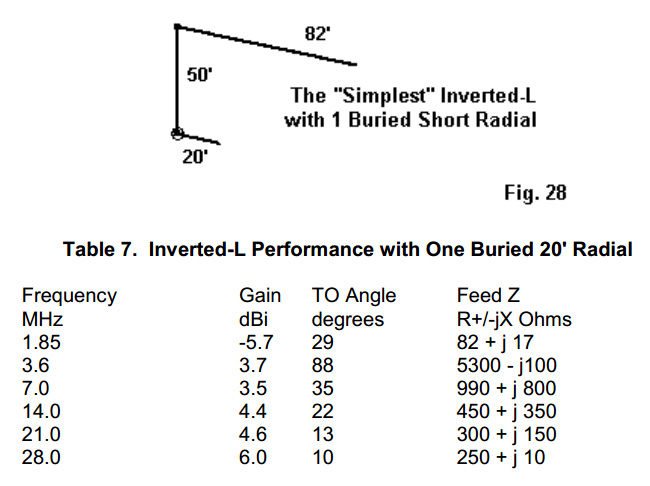
Table 7. Inverted-L Performance with One Buried 20′ Radial
Frequency Gain TO Angle Feed Z
MHz dBi degrees R+/-jX Ohms
1.85 -5.7 29 82 + j 17
3.6 3.7 88 5300- j100
7.0 3.5 35 990 + j 800
14.0 4.4 22 450 + j 350
21.0 4.6 13 300 + j 150
28.0 6.0 10 250 + j 10
As Table 7 shows, performance does diminish relative to the other systems. However, only on 160 meters, where we lose another 3 dB of gain, is the result unworkable. On the other bands, we obtain usable performance. For emergency and field operations, the 1-radial inverted-L may be usable from 80 through 10 meters. However, for home use, we should strive to add as many radials shaped however the ground will permit–as we can, even if weonly add one every few months. The usual safety precautions apply to radials: get thembelow ground where playing children, gardening spouses, and seeing-eyelawn mowers cannot reach them. If you usea tree to support the feed-end of the antenna, be
sure to space the vertical well away from the tree trunk. In addition, make sure that no one can touch the antenna or its feedpoint during operation.
You will note that the 80-meter impedance is very high, higher than most antenna tuners can handle.For this reason, many inverted-L users prefer to use wire lengths longer or shorter than the 130′ length that I used in this demonstration. A 3/8-wavelength inverted-L (about 100′ including both the vertical and horizontal sections) will move the very high impedance frequency to the 30-meter band. Pattern shapes will change, but the general properties of the inverted-L will remain: good (but not great) bulbous patterns for general communications in almost every direction. One final question: where do I place the antenna tuner? The answer is simple: at the antenna feedpoint. This position is standard in the field, where we usually terminate the antenna at the operating position with a manual antenna tuner. For this antenna, we actually need a single-ended network tuner. At home, the inverted-L is an ideal application for one of the weather protected automatic tuners (using precautionary additional weather shielding) with the case or ground lead connected to the radial side of the system.
The advantages of this type of system are obvious: automatic (or semi-automatic) tune-up with a coaxial cable from the antenna feedpoint to the rig. The disadvantages are the initial expense of the automatic tuner and periodic preventive maintenance. Final Notes: We have now surveyed my personal five favourite multi-band wire backyard antennas. There are others that I might have included. In fact, I thought of some others, but gradually discovered that they were mostly variations of the ones that I included. For example, there are some sloping and bent wire antennas calling for either measured or random-length “counterpoises.” However, they are simple variations on the inverted-L. Linear dipole-doublets have inverted-Vee variations. I have omitted antennas using traps, simply because traps require maintenance and represent an advanced project for most folks who roll their own.
All of the antennas I chose involve only wire and feedline plus, of course, the antenna tuner. For all but the inverted-L (and possible the fanned dipoles), we need a balanced tuner that will handle a very wide range of resistance and reactance at its terminals with the highest possible efficiency. Although there are a few balanced network and Z-match tuners available, most hams still use single-ended network tuners with a 4:1 balun at the terminals. Unfortunately, not all 4:1 tuner baluns are made equal, and many show high losses in the presence of either high reactance values or very low impedances that may occur as the feedpoint impedance is transformed along the parallel feedline. There is an alternative system for using the single-ended network tuner in the manner in which it is most efficient: as a single-ended network. Fig. 29 shows the essentials.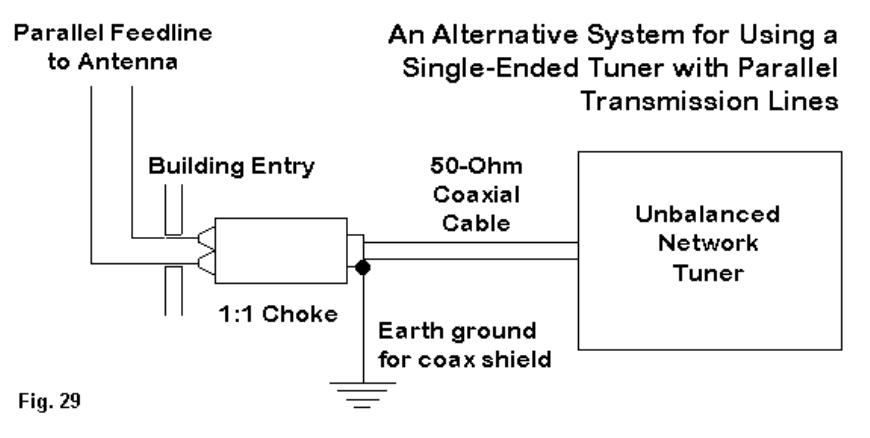
At the shack entry point, we terminate the parallel feedline with a 1:1 balun.Actually,the unit is a simple choke in preference to a transmission-line transformer that prefers a minimum of reactance. A W2DU type choke composed of about 50 ferrite beads around a length of coax tends to work quite well in this application. We run a lead to an earth ground from the coax braid right at the coax side of the choke itself. This measure tends to attenuate any remnant RF that might get onto equipment cases or into circuitry. Make the coax run as short as possible using the lowest-loss coax that you can obtain, since there will still be a considerable SWR on the line to the tuner. However, this line goes directly to the coax connector on the tuner output side for single-ended processing. The system is not perfect and does have small losses. However, in most cases, it tends to clear the shack of unwanted stray RF from indoor parallel feedlines, and it does allow the single-ended tuner to effect a reasonably efficient match with the remainder of the antenna system. This system is not new,being almost as old as the W2DU type choke itself. I first recommended it as one solution to problems some folks had back in 1980 with G5RV antenna systems.
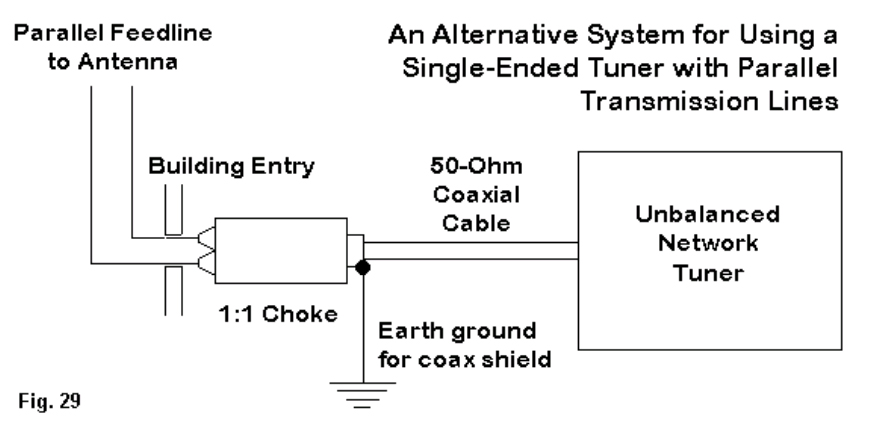
There is a vast territory that these notes have not covered. We can make multi-band beams, multi-band verticals, and a number of other antennas that will cover 2 or more of the ham bands. I encourage you to experiment with antennas, since wire is inexpensive and you can develop temporary mounts to put up and take down your trial antennas. But when you do erect an antenna, please be sure to give it that same care that you give your transceivers. Periodic preventive inspection and maintenance will ensure that the antenna gives you all the performance that it can every time you fire up the rig. Attention to safety will protect both property and the lives of those you love the most including yourself.
As for those other possible antennas, there are always future FDIM celebrations to cover them.
73 L Cebik W4RNL